Science – Overview
Science for tomorrow's reality
For decades, the natural sciences were characterised by the study of ever smaller elements in nature. Research focused on identifying these details and exploring their properties. For some years now, the focus has been shifting: the interaction of the individual elements is increasingly the focus of interest. Because this is the only way to describe the dynamics and functions of complex systems: From the multi-body systems in the atomic nucleus to the outstanding performance of the human brain.
FIAS sees itself as a platform for theoretical research into complex self-organising systems of nature. Experimental data can often only inadequately describe the interaction of the components. Here, sound theoretical foundations and analyses are becoming increasingly important. At FIAS, these are created through mathematical algorithms and simulations, but above all through cooperation across disciplinary boundaries.
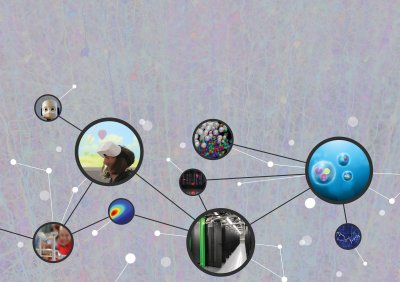
Research Areas
Theoretical Sciences
Theoretical physics is the discipline that aims at describing how the world works in terms of fundamental equations. The goal is to abstract explicit phenomena by reducing them to underlying principles that are responsible for many different manifestations in nature.
Bio- and Neurosciences
The modern life and neurosciences are increasingly characterised by mathematical and quantitative approaches - in no other discipline are the challenges to theory, modelling, and simulation growing so fast.
Computer Science
Within the last decade high performance computers have become an integral part in todays science and society. Most simulations and experimental analysations of complex systems require a high amount of computing power.